Research
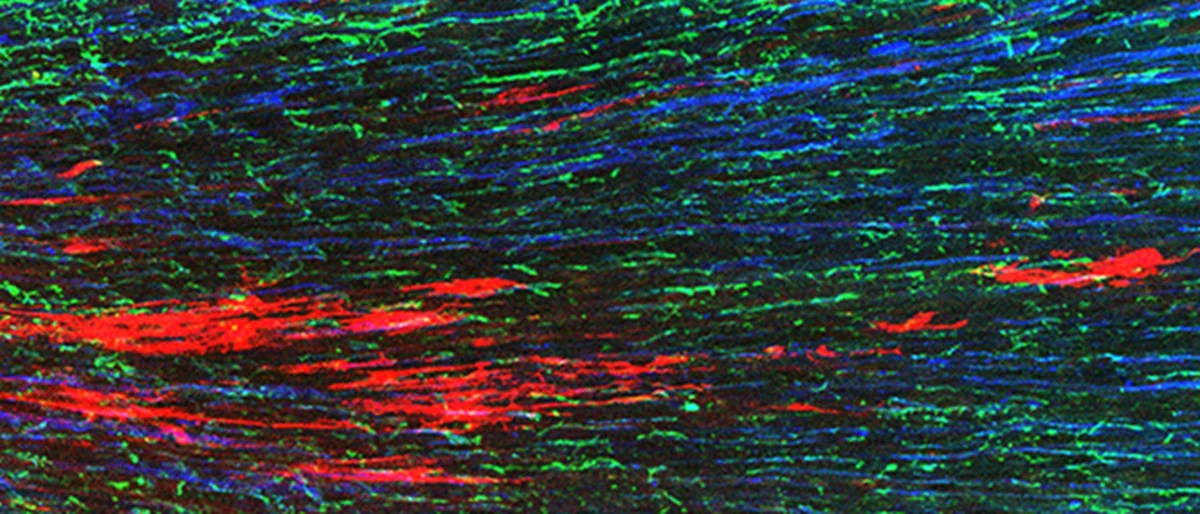
The Butler laboratory investigates the mechanisms that establish sensory relay circuits during embryonic development, to then recapitulate these processes to regenerate neural circuits damaged by either injury or disease. Sensory relay circuits arise in the dorsal spinal cord, transmitting sensory information received in the periphery to higher-order centers in the brain, or spinal motor circuits. They encode critical, but often overlooked, sensory modalities, including proprioception, nociception, and touch. These modalities are central to the human experience, allowing us to hold our bodies in space, react to pain, and interact with our environment.
We study first, the molecular mechanisms that establish the distinct fates of different classes of dorsal interneurons (dIs) in the developing spinal cord. Our studies have focused on three key instructive growth factors, the bone morphogenetic proteins (BMPs), the Wnt family and retinoic acid (RA), which together direct the formation of six populations dIs, dI1-dI6. The dIs encode different sensory modalities, including proprioception (dI1), motor output (dI2, dI3, dI6), nociception (dI4, dI5) and touch (dI5). We have leveraged our understanding of cell fate specification to establish the first directed differentiation protocols for dIs from human and mouse pluripotent stem cells (PSC). Our recent studies have defined two protocols, which derive stem cell-derived dIs that are transcriptionally indistinguishable from their in vivo counterparts and exhibit the correct sensory function signatures. These stem cell models represent a new model system for studying spinal cord development, as well as permitting us to work towards a cellular replacement therapy that will restore sensory function in spinal injury models.
Second, we study the signals that direct dI axons into their initial circumferential trajectory in the spinal cord. Canonically, these signals were hypothesized to act over long distances, however, our recent studies have revealed the central importance of short-range signaling in axon guidance. We focus on understanding the role of netrin1, which was first identified 30 years ago. Our studies have unexpectedly found that netrin1 can function as a novel directional adhesive (haptotactic) cue, which regulates the shape of commissural (dI1) axon growth in the spinal cord. We are continuing to resolve the role(s) of cell-type specific netrin1; this understanding will be critical to correctly deploy netrin1 for neural repair.
Third, axon guidance studies have historically focused on identifying molecules that provide spatial cues to axons. However, for neural circuits to form in synchrony within the developing embryo, axons must reach directional information at particular times in development, suggesting the existence of temporal guidance signals. We identified the first temporal guidance cues in the spinal cord, when we showed that the BMPs control the rate of dI1 axon growth away from the dorsal midline. We are currently assessing whether this mechanism that controls axon growth rate, can be co-opted to accelerate regenerating peripheral nerves. These studies have the potential to reduce painful recovery times for nerve-injury patients.